Biomaterials are at the forefront of important advances in health sciences. Given the aging population and the growing number of chronic illnesses, which represent major challenges for the public health sector, the demand for more sophisticated medical products is expected to increase in the coming years, hence the need for ongoing research into state-of-the-art technology. Biomaterials offer a wide range of opportunities to address these issues. As their name indicates, biomaterials are the result of a synergy linking several disciplines, notably material sciences and biology, as well as a number of new techniques in biomechanics, biophysics and biochemistry.
Derived from natural or synthetic sources, biomaterials are used in medical devices that interact with biological tissues. These include supporting tissues, such as skin, bones and teeth, as well as blood, which is made up of several types of cells (red and white blood cells) and various substances. Biomaterials can be composed of metal and metal alloys (stainless steel, titanium, etc.), ceramics (alumina, phosphate, etc.), polymers (functional or resorbable) or naturally sourced materials (biological tissues, chitin, cellulose, etc.). Examples of these applications are numerous: heart valves, orthopedic prostheses, hydrogel contact lenses, implants and so forth. The table below contains some examples of how this technology is utilized:
Medical Field |
Applications
|
Cardiovascular treatments
Orthopedic surgery
Ophthalmology
Other |
Pacemakers, vascular prostheses and endoprostheses, catheters, heart valves, stents
Orthotics, hip implants, prosthetic tendons, bone replacements and fillers, bone fracture repairs, etc.
Implants, contact lenses
Biosensors, controlled drug delivery systems, portable and implantable pumps |
Despite many noteworthy advances in recent years, conventional biomaterials continue to pose multiple challenges, among them immune intolerance, infection, inflammatory reaction and incompatibility. Research is key to overcoming these difficulties and improving the outcomes of medical treatment. The goal of ongoing efforts in this regard is to ensure the safety and efficacy of biomaterials used in the human body. They must therefore meet biocompatibility requirements, which refer to the capacity to deliver an appropriate response in a specific situation, without any adverse effects on the host environment. In other words, the body must tolerate a biomaterial’s components and the products into which it breaks down without inflammation, infection or rejection (immune intolerance).
New biomaterials are making a name for themselves in such fields as tissue engineering and regenerative medicine, early medical diagnosis, cardiovascular treatment, nanosurgery, cell therapy and even targeted drug delivery into biological tissue. Emerging developments include new hydrogels, smart stem cell-based materials and biodegradable dental resins.
The purpose of this report is to provide an overview of biomaterial technologies that have recently become available or are currently being developed in Montreal. The first part of the report summarizes the worldwide market for biomaterials and emerging biomedical applications. The second part explores a number of leading-edge technologies developed by Montreal-based researchers and companies. Note that this is not intended to be an exhaustive compendium. The conclusion provides a general review of the contents of the report and stresses the important role of biomaterials in our demographically changing population.
THE MARKET FOR BIOMATERIALS IS EXPLODING, THEREBY STIMULATING THE TISSUE ENGINEERING AND DRUG DELIVERY SECTORS
- Biomaterials
The worldwide biomaterials market was estimated at $62 billion in 2015, compared with $48 billion in 2013. This translates to an average annual growth of about 14% within this period. Between 2016 and 2021, it is expected to increase by 16% per year on average to top the $149-billion mark in 2021. Valued at $22.75 billion, the North American market made up a 37% piece of the global pie in 2015. This will likely ease off slightly in 2020 to about 34% of the worldwide total. This sustained growth has trickled over to a number of emerging applications, such as targeted drug delivery and human tissue regeneration.
- Advanced drug delivery systems
In 2015, the global market for advanced drug delivery systems was estimated at roughly $179 billion, compared with $151 billion in 2013. This represents an annual average growth rate of 9%. In the coming decade, the industry is expected to expand at a rate of 6% per year to reach close to $331 billion in 2025. The North American and European markets are the primary drivers of this boom. In 2015, they were together responsible for a full 74.4% of worldwide revenues. This percentage should remain high in the years to come, hitting 73% in 2020.
- Tissue engineering and regenerative medicine
The global market for tissue engineering and regenerative medicine was valued at $27 billion in 2015, averaging 27% yearly growth between 2013 and 2015. This trend should continue for several years to come, taking the market from $32 billion in 2018 to $135 billion in 2024. Growth in this industry is powered by the North American and European markets, which together accounted for 83% of the international market in 2014. This proportion will remain high going into 2019 at 80%.
This industry is faring particularly well in advanced economies, specifically North America and Europe. Analysts point to factors such as the aging population and longer overall life expectancy as being key drivers of the growth in the biomaterials market. Also stimulating market growth are pro-clinical trial government policies and the availability of research funding.
MONTREAL: A FERTILE BREEDING GROUND FOR BIOMATERIALS DEVELOPMENT
Given their biocompatibility, biodegradability and inorganic properties, biomaterials are an increasingly popular subject of leading-edge research and development efforts. Montreal-based businesses and scientists are making their mark in several promising areas, such as drug dosage and targeted drug delivery, enhanced tissue engineering and minimally invasive treatments.
- Sophie Lerouge | Hydrogels and bioactive coatings for minimally invasive therapies
Sophie Lerouge, a professor at the École de technologie supérieure (ÉTS), the Chairholder of the Canada Research Chair in Biomaterials and Endovascular Implants and a researcher with the Centre de recherche du Centre hospitalier de l’Université de Montréal (CRCHUM), develops innovative biomaterials to tackle an array of clinical challenges. In her work, she endeavours to make therapies less invasive and more precisely targeted to reduce the cost and complications associated with conventional surgical treatments.
Her research, which is being carried out in close collaboration with clinicians and the biomedical industry, focuses on both the creation of new coatings and the development of hydrogels. The purpose of bioactive coatings, which are produced by plasma processes and the chemical grafting of biomolecules, is to modify a material’s surface in order to optimize the human body’s biological response. The findings of this research thrust are paving the way to a new generation of medical devices, such as endoprostheses, metallic stents and vascular prostheses used in the treatment of heart disease. These bioactive coatings can be used with traditional implants, helping to improve their efficacy and minimize complications without altering their volume or longevity, thus facilitating the technology transfer process.
With injectable hydrogels, which represent a significant part of her work, she aims to develop and optimize cell therapy and biological tissue regeneration. Designed to counter side effects and reduce the invasiveness of surgical procedures, these hydrogels are beneficial for several reasons. They are thermoresponsive, taking on a liquid form at lower temperatures and a solid form at body temperature, and therefore easy to inject and mechanically cohesive once they have gelled. Furthermore, they are biocompatible and allow for an excellent rate of cell survival within the gel.
These innovative hydrogels make it possible to release molecules or cells in a targeted way at the local level. In addition, they act as a matrix for tissue regeneration. They are intended primarily as an enhanced solution for treating diseases such as cancer and the degeneration of cardiovascular and orthopedic tissues.
As an example, Dr. Lerouge has developed a biogel in which a patient’s immune cells can be introduced to treat cancer at the local level. Made from chitosan, which comes from crustacean shell powder, this biogel is a liquid at room temperature and a gel at 37°C. It is therefore easily injectable via syringe or catheter straight into a tumour site. The immune cells grow in this environment and can exit the gel to attack the tumour. Her work, which is being conducted in collaboration with Université de Montréal researcher Réjean Lapointe, represents an initial step forward in localized immunotherapy, which holds a clear advantage over conventional treatments, where the drugs used affect the entire body, generating adverse side effects and higher costs.
Through the technological advances being made by her research chair, Dr. Lerouge has forged meaningful ties with the biomedical industry in the local, national and international arena.
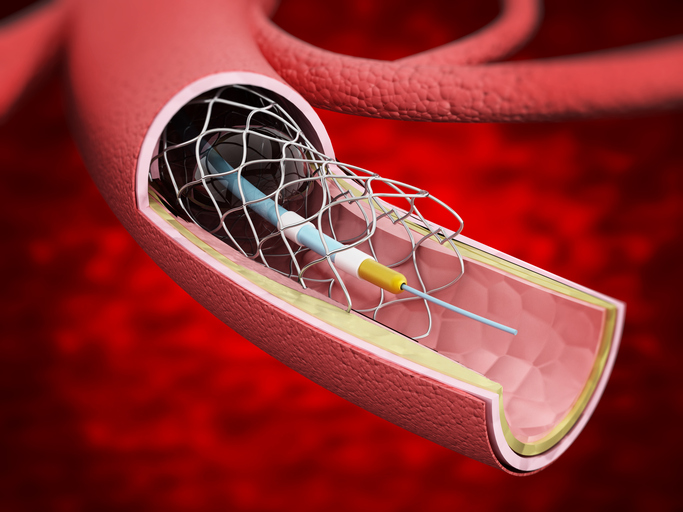
Detailed illustration showing vascular stent inside the vein.
- Julian X. Zhu | Bile acid-based biomaterials for biomedical and pharmaceutical applications
Professor Julian X. Zhu, the Chairholder of the Université de Montréal Research Chair in Polymer Biomaterials, focuses his research on the development of polymer biomaterials for biomedical and pharmaceutical applications. More specifically, he develops biodegradable polymers, next-generation dental resins, hydrogels and biosensors. These biomaterials are made using natural composites, including bile acids, a family of biocomposites that are plentifully available in the human body, not to mention non-toxic and biocompatible. For these reasons, Dr. Zhu believes they are good candidates for biomedical applications.
He has patented a number of cutting-edge technologies, including dental resins that, unlike other resins on the market, are biocompatible and non-toxic and exhibit beneficial mechanical properties. Conventional dental resins contain bisphenol A (BPA), an organic substance that is used in preparation of certain plastics and that is toxic for human health and the environment. BPA is a known endocrine disrupter that causes cardiovascular and reproductive problems as well as some types of cancer. The development of dental resins using biocompatible substances would help prevent these health risks.
Another promising and patented development for Dr. Zhu and his team has been biodegradable polymers. Using bile acids, they have devised biocompatible polyesters with a controllable degradation rate. The results of this research may lead to a number of biomedical products, including bandages for tissue-engineering applications, biodegradable polymer stents whose shape can be controlled during surgery and smart sutures.
Dr. Zhu has also developed innovative hydrogels made of glucose and bile acid that exhibit good biodegradability and functional diversity. The benefits of these new aqueous materials that promote self-healing would be enormous for the medical community. These hydrogels foster the automatic regeneration of soft tissue. Examples of their use include self-healing sutures and targeted drug delivery.
These biomaterials, which comprise only a portion of Dr. Zhu’s research, have yet to be commercialized. Industry partnerships would help bring these products to market more quickly.
- L’Hocine Yahia | Superparamagnetic nanoparticles and bacteriophages for nanomedicine applications
The development of a nanoscale drug delivery system represents a major challenge due to the dispersion of nanoparticles in biological cells. L’Hocine Yahia, head of Polytechnique Montréal’s Laboratory of Innovation and Analysis of Bioperformance, affiliated with the Université de Montréal’s Faculty of Medicine, develops smart biomaterials that may have a major impact on cellular response. His two main research thrusts right now are focused on designing a superparamagnetic nanoparticle platform and developing methods to destroy the antibiotic-resistant bacteria responsible for nosocomial infections. These infections occur during the delivery of medical care in a health care institution and affect patients and caregivers alike.
A superparamagnetic nanoparticle platform will have various applications in the field of nanomedicine. More specifically, Dr. Yahia’s research will enable two key applications related to medical targeting within the body:
– Targeted injection of drugs into tumours
– Capture of specific pathogens and proteins in the blood
A magnetic field raises the temperature of the nanoparticles and causes them to give off heat (hyperthermia). These heated nanoparticles then destroy the cancer cells in any tumours that they come in contact with. In addition, research in this area is being used to increase the capacity of materials to react to a magnetic field in order to release more heat and reduce the number of nanoparticles injected into biological cells. These superparamagnetic nanoparticles will make it possible to develop new methods to prevent and treat diseases such as atherosclerosis, cancer, cholera and malaria.
One of the main challenges related to nosocomial infections is bacterial resistance to the antibiotics currently used to prevent and control them. Research and development therefore represent an important step forward in confronting this problem. Accordingly, the work currently underway at the LIAB focuses on two methods:
– The first method, which is based on metal nanoparticles, makes it possible to generate reactive oxygen species (ROS).
– The second uses natural viruses (or bacteriophages), which infect bacteria but ignore everything else. The performance of medical devices such as prostheses and implants is highly contingent on the characteristics of the biomaterials used. Nosocomial infections can be transmitted through these devices. The method proposed by Dr. Yahia and his team involves attaching bacteriophages to the surface of implants and medical devices to prevent the growth of microbial biofilms that cause infection.
This research will eventually lead to the development of medical nanodevices used to prevent and treat diseases such as cancer, atherosclerosis and the nosocomial infections that have become a major health concern in recent years.
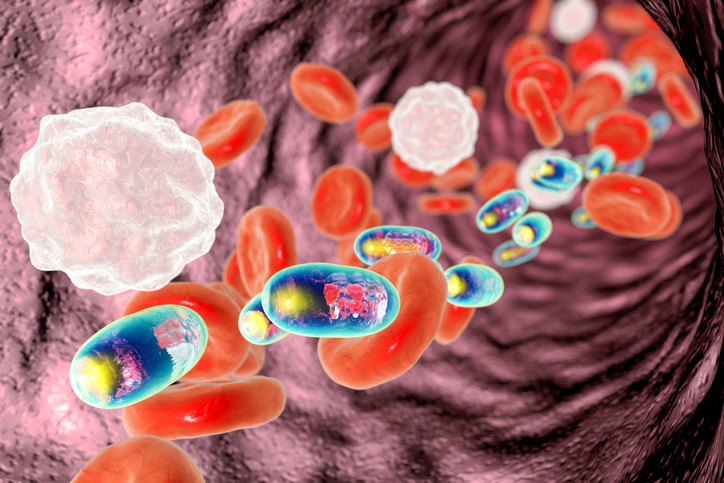
Delivery of medicines inside polymer nanoparticles
- Jung Kwon (John) Oh | Smart delivery nanoassemblies for enhanced cancer therapy
During chemotherapy, a dose of an anticancer drug is administered intravenously into the blood. Ideally, the drug should be administered at exact therapeutic concentrations and targeted to tumours to kill only the cancer cells. However, the delivery of small-molecule drugs to tumours is often not well controlled, thus causing severe side effects as well as poor drug efficacy. These negative results are mainly attributable to the small size of the molecules. They are rapidly filtered by the kidney for elimination from the blood and they are also distributed to normal tissues.
In order to improve the delivery of small-molecule drug therapeutics, nanoscale platforms, whose sizes range from 10 to 100 nanometres, have been developed for tumour-targeting delivery applications. A promising platform is the polymer-based nanoassemblies designed to an appropriate size (50–150 nanometres in diameter) that can maximize drug efficacy and minimize the undesired side effects common to small-molecule drugs. These nanoassemblies enable the encapsulation of anticancer therapeutics to function as drug-delivery nanocarriers (or nanotherapeutics). Upon intravenous injection into the bloodstream, the drug-loaded nanoassemblies (or nanocarriers) are circulated in the blood. Through a process known as the enhanced permeability and retention (EPR) effect, they target tumour tissues only and are then internalized into cancer cells through endocytosis (a process by which the cell takes in molecules through its membrane). As a consequence, these drug-loaded nanoassemblies minimize the undesired side effects common to small-molecule anticancer drugs and maximize the drug efficacy.
However, a critical challenge is that the nanocarriers have to undergo a sustainable release of therapeutics inside the cancer cells and tumour tissues. One innovative solution is presently being developed by Professor John Oh, the Chairholder of the Canada Research Chair in Nanobioscience, and his team at Concordia University. Oh’s research focuses on the design and processing of polymer-based nanomaterials that hold great potential as imaging agents, tissue-engineering scaffolds and, particularly, drug-delivery carriers that target tumours. More specifically, with his research group, Oh is studying stimuli-responsive degradation in order to control and enhance the release of drugs from drug-loaded nanoassemblies. Stimuli-responsive degradation involves the incorporation of cleavable linkages into the design of block copolymers and their assemblies. They are designed to be stable under physiological conditions while circulating in the blood. However, they are disassembled in a controlled fashion as cellular components to give the appropriate stimuli inside the tumour and cancer cells. Such disassembly enables control and enhancement of the release of the encapsulated drugs inside the cancerous cells and tissues. They have put significant efforts into the development of a variety of novel stimuli-responsive degradable block copolymers and their nanoassemblies. The developed nanotherapeutics interface with biological processes, and an understanding of their biological functions offers enormous potential for cancer research and treatment.
In addition to the block copolymers and their assemblies, Oh and his team have developed a variety of reduction-responsive nanomaterials, including nanofibres, thermoresponsive polymers and nanogels, for a wide range of biomedical applications. Overall, their research offers great potential for enhancing the quality of life for cancer patients by improving the efficacy of the treatment.
- Mircea Alexandru Mateescu | High-potential therapeutic applications
Targeted formulations
Histamine is an allergen and inflammatory agent found in a number of pathologies, including allergies and inflammatory bowel diseases. Instead of synthetic antihistamines and anti-inflammatories, diamine oxidase (or histaminase), an enzyme of vegetable origin, could act on excess histamine and thus promote better intestinal mucosal health. To remain active and fulfill this role, the enzyme must be made up of excipients that are also non-toxic. In this context, the team working with Mircea Alexandru Mateescu, a professor of chemistry at the Université du Québec à Montréal specializing in the field of protein biochemistry and pharmaceutical applications, has proposed a therapeutic solution using targeted biopolymer-based formulations. These help ensure the drug’s stability and facilitate the release of diamine oxidase within the inflamed bowel. Note that this enzyme and the excipients in the formulation are sourced from non-toxic food constituents.
Another promising initiative being undertaken by Dr. Mateescu’s team is the in vivo development and monitoring of oral formulations targeting mucosal immunity using barium sulfate as a tracking agent. This initiative, led by Marc-André Labelle, a graduating master’s student in biochemistry, aims to devise a new method for monitoring oral formulations containing the fimbriae peptide (F4) for local drug delivery in the small intestine. This involves incorporating an opacifier known as barium sulfate in the tablets to be administered. The barium sulfate makes it possible to follow the tablets’ path and confirm that the bioactive agents have been delivered to their target to achieve the desired outcome. The in vivo monitoring itself will be performed using X‑ray technology. According to the young scientist, this technique is more efficient than a bone scan and less costly than an MRI.
Cell cultures
For people suffering from chronic diseases affecting the liver (hepatitis B, C or D) or pancreas, organ and tissue transplants are often the elected course of treatment. For example, a liver transplant, a surgical procedure to replace a diseased organ with a healthy one, is necessary to treat patients suffering from liver failure. This preferred option is nevertheless limited by certain factors, not the least of which is the shortage of donors. In this context, Dr. Mateescu’s team is currently conducting research to find alternatives to organ transplants by creating organs and tissues from stem cells. They have developed biomaterials with mechanical, non-toxic properties that allow the growth of three-dimensional tissues. More concretely, they have devised a new polyvinyl alcohol (PVA) derivative as a scaffold for growing hepatocytes in a 3-D microenvironment. In addition to regenerating liver tissue, these hepatocyte scaffolds can be used to assess various drugs and toxic agents.
Another member of Dr. Mateescu’s team, Nassim Benyerbah, a master’s student in biochemistry at the Université du Québec à Montréal, is working to develop a 3-D biocompatible and biodegradable material from starch and PVA derivatives for various biomedical applications such as cell cultures and implants. The goal is to produce biomaterials in sponge form with a 3-D scaffold structure composed of interconnected pores that can host cells and offer conditions conducive to their proliferation.
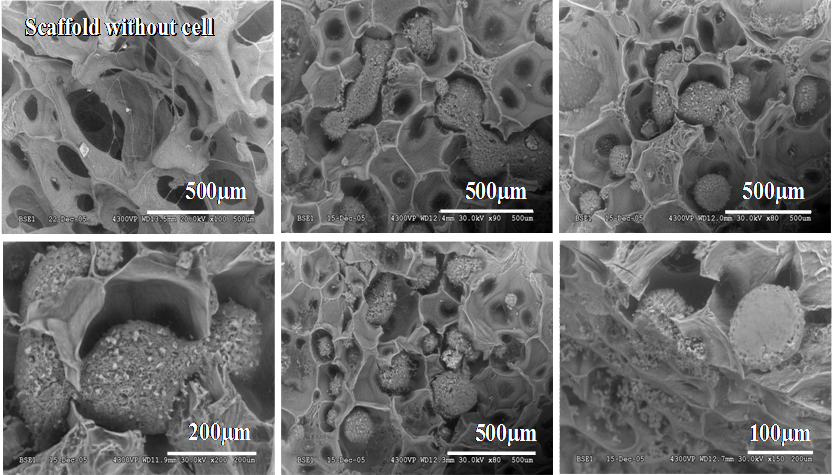
Scanning electron microscopy for PVA-STMP-CMS-based carriers with and without hepatocytes (48h). Images obtained at an accelerated voltage of 15 – 30 kV and a magnitude of 100-500 μm. | Image credit: Prof Mircea Alexandru Mateescu
- Bioastra Technologies | Smart, leading-edge materials
Montreal-based Bioastra Technologies specializes in intelligent, adaptive materials. Building on its multidisciplinary scientific expertise in polymers, chemical engineering, textiles, surface science and biomedical engineering, the company has positioned itself as a world leader in its field.
Bioastra develops smart polymers that can change their intrinsic properties when they encounter an external stimulus, such as stress, temperature, humidity, pH, electrical fields and magnetic fields. These materials have multifunctional properties and can be used in a wide range of applications, including medical devices. Among the products developed by the team of young researchers at Bioastra are thermoresponsive sol-gel materials for embolization and drug delivery. These materials can transform from a solution (“sol”) to a gel. This entails certain physical changes as they transition from a mostly viscous liquid to a mostly elastic, structural solid (with a different porosity).
The research team is also involved in other activities, such as the development of flexible polymer sensors and artificial muscles using electroactive polymers. This type of polymer is unique in its ability to convert electrical energy into movement and force, and vice versa.
- Oligo Medic | A cutting-edge solution for patients with advanced cartilage loss
Joint pain is often attributable to worn cartilage, the tissue that connects bones together for the primary purpose of promoting joint mobility and flexibility. Unlike bone, cartilage is vulnerable and does not regenerate or heal easily. It is often exposed to diseases that can cause irreversible tissue damage. Osteoarthritis is a good example of a degenerative condition that attacks articular cartilage. Mainly targeting the elderly and young athletes, osteoarthritis can completely erode cartilage and cause intense pain. Until now, the solutions offered to patients have been joint replacement surgery and pain-alleviating drugs.
To address this problem, Oligo Medic, a company specializing in novel biomaterials, developed and patented an innovative product that regenerates cartilage. Known as JointRep, it is a polyglucosamine-based hydrogel with thermogelling properties. It solidifies quickly after injection as it reaches body temperature. One of the main advantages of this product is its biocompatible, isotonic and bioadhesive matrix.
The medical device is administered percutaneously, via arthroscopy, under local anaesthetic. A miniature fibre-optic camera and a tube through which various tools are introduced are both inserted into the knee. The damaged cartilage is smoothed out and holes are drilled into it to release stem cells from the bone marrow. The hydrogel is applied to the cartilage and, once exposed to body temperature, transforms into a solid gel scaffold around which the stem cells can produce new, healthy cartilage. Only 90 minutes after the procedure, the patient can be discharged from the hospital, walking with a cane. Regular activities can be resumed within three weeks.
Clinical trials have been conducted in Europe with patients 18 to 75 years of age suffering from advanced cartilage loss. Subjects were selected for standard microfracture treatment or microfracture treatment using JointRep. After six months, the results for the group treated with JointRep hydrogel were conclusive. The product helped reduce pain and increase physical dexterity and mobility in every age group.
JointRep has received CE marking from the European Economic Area for use on all joints. It is sold in 15 countries around the world, although it is not yet available in North America. Efforts are currently underway to obtain approval from the U.S. Food and Drug Administration.
It is important to overcome the challenges inherent in demographic changes and the increased prevalence of chronic disease with technologies that are more and more precise. In every medical field (surgery, tissue engineering, biotechnology, etc.), developing and disseminating efficacious health care technologies are among the preferred avenues to be explored in this regard.
The Montreal-based technologies presented in this report speak to the strong potential that biomaterials represent for the life sciences sector and are indicative of the overall openness toward new biomedical applications. Regenerative medicine and targeted drug delivery are two areas that seem particularly promising. Given the ever-changing nature of these industries, especially the emergence of new technologies such as 3-D bioprinting and high-performance materials, they are likely to play an increasingly important role in the years to come. They are opening the door to many new opportunities in Montreal, a city already known for its innovation in health care technologies, biopharmaceutics and life sciences in general.
Montreal ranks seventh worldwide for its concentration of jobs in this key sector and possesses a critical mass of health sciences companies of all sizes. Transferring the technology outlined in this report is vital to fostering vitality and innovation in this industry and promoting the city’s reputation for excellence.